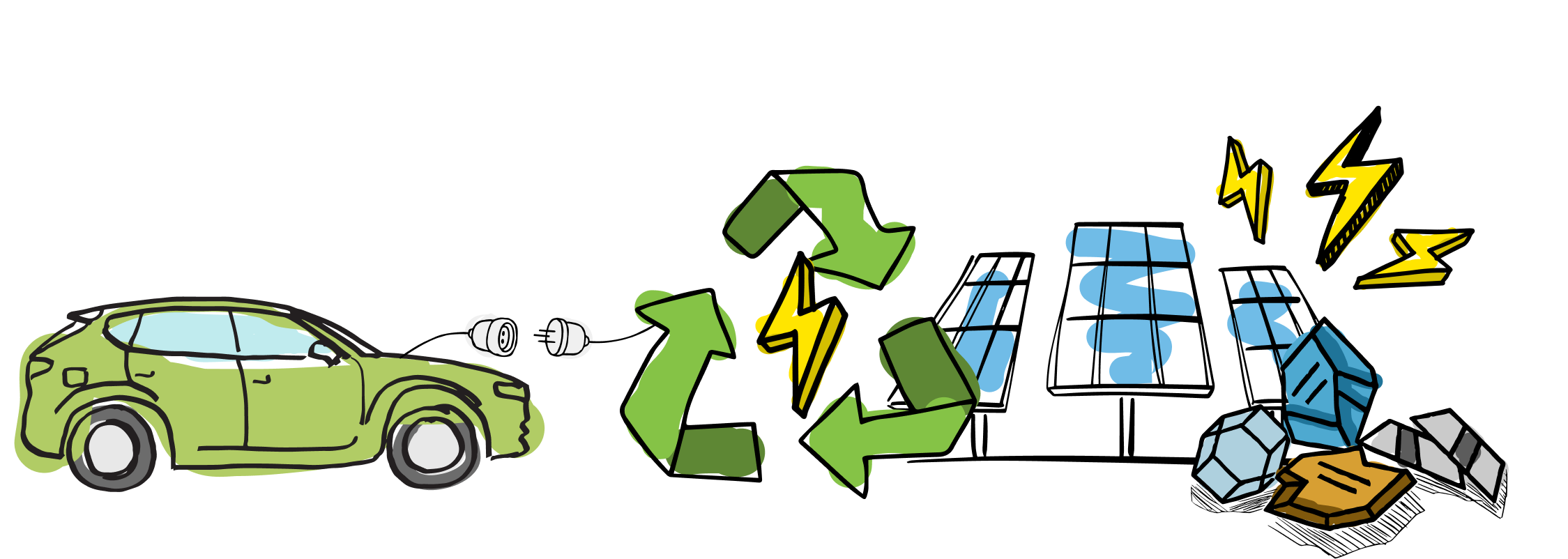
An electrified economy is a more circular economy.
This might sound counterintuitive to some. Electrification involves replacing millions of fossil machines with electrical equivalents, like solar panels and batteries, which eventually reach end-of-life too. Surely, this poses major challenges for waste management?
It doesn’t have to be this way, because an electrified energy system is actually full of opportunities for redesign, reuse, recovery and recycling. There are opportunities for innovators and entrepreneurs to generate value across the lifespan of every energy asset, not least the return of its components to the manufacturing cycle.
To explore this further, Rewiring Aotearoa has partnered with Circularity, local experts in circular design and strategy, to explain what the circular economy is, how to integrate circularity into the design of energy systems, and the role of policy strategy to make it happen.
We show that an unmitigated flood of e-waste is avoidable, unlikely and actually in our interests to avoid. If we, as a country, can’t get our act together to recover valuable metals and minerals for future manufacturing, then clean-tech companies won’t want to export those precious resources to us. They need those resources back, so countries that send e-waste to landfill run the risk of being shut out of technology markets, unable to embrace the energy transition.
So what can we do to embrace the opportunities of the circular economy?
In this piece, we explain:
- The circular economy
- Closed loop systems
- Smart materials
- Circular business models
- Regenerative behaviours
- Network Participation & Embedded Intelligence
- Where to from here?

Let's square that circle!
What actually is the circular economy?
In a linear economy, we take, we make and we waste. We extract virgin, often non-renewable, resources from the earth to produce goods and services. Afterwards, we discard these products as waste.
By contrast, a circular economy is shaped by three principles [1]:
- eliminate waste and pollution;
- circulate products and materials; and
- regenerate nature.

This creates closed loops of materials which extend their use and re-use, reducing our need to extract new materials from the earth [2].
Yet while the rhetoric on circularity has increased exponentially over the last few years, the rate of resource reuse in the global economy is actually declining. About 100 billion tonnes of resources are used annually, yet the share of secondary materials was only 7.2% in 2023, down from 9.1% in 2018 – a 21% decline in five years [3].
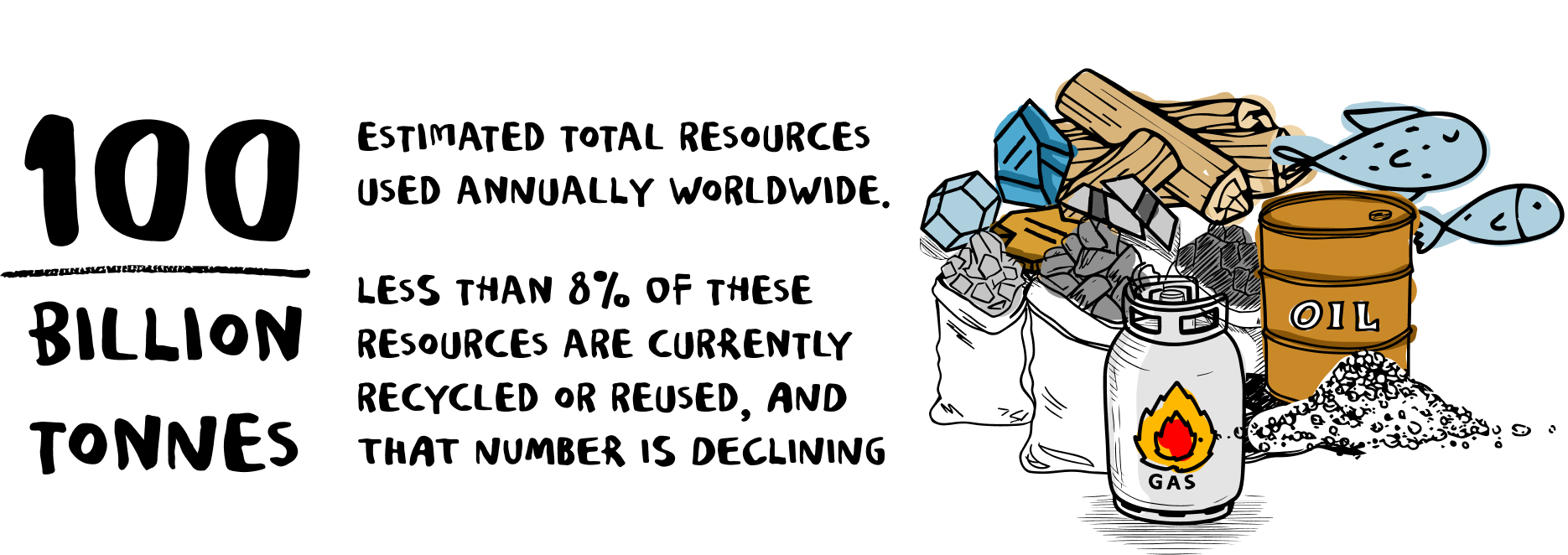
The fossil energy system is, by and large, a linear economy. As discussed in a previous explainer, we currently shift over 15 billion tonnes of coal, oil, and fossil gas around the world every year. Much of this becomes waste, both lost as heat during combustion and dumped into the atmosphere as carbon dioxide (CO2) emissions. Other waste flows also proliferate, such as the 1.1 billion tonnes of highly toxic coal ash produced globally every year [4], some of which is diverted into road construction, but most of it discarded with serious environmental hazards [5].
Not only are the potential waste flows from renewable energy much smaller [6], the opportunities for circularity in renewable energy technologies are much more plentiful. The recovery rates of materials in solar panels are already well over >90% and, as we’ll discuss later, this can be further improved through better design [7]. Understanding this is critical, not only for reducing environmental impacts, but also addressing barriers to the public acceptability and social licence for electrification.
To unpack the opportunities for a circular energy transition in Aotearoa New Zealand [8], we use Circularity’s circular-by-design methods and several case studies:
- Closed loop systems to keep materials in use
- Smart materials that are derived from biomaterials
- Circular business models that create extra value from resources
- Regenerative behaviours that sustain precious resources
- Network participation and embedded intelligence to enable scaleable, smarter systems
Closed loop systems
Closed loops are a key concept in the circular economy. The aim is to ensure that materials in a product are kept in use by designing in repair, recovery and reuse from the outset. By designing closed loops to keep materials in circulation, we reduce the need for new raw materials and cut down the waste.
In the energy transition ahead, there are two loops to close. First, the flow of new materials being mobilised by the energy transition, such as the lithium in batteries and the many metals in solar panels. Secondly, the flow of existing materials in fossil-fuelled machines that are poised to enter landfills once they reach end-of-life, or retired early to make way for electrical alternatives.
The mismanagement of either of these material flows could result in unnecessary waste. In turn, this could produce a public backlash against the energy transition and its impacts. Nobody wants an energy transition that litters the landscape with old petrol cars, unusable heaters, and other defunct machines. But by creating closed loops, we channel these materials back into the manufacturing cycle.

In a world of closed loops, the old fossil system can be ‘mined’ by the new energy system, a reservoir of useful materials that can be extracted and repurposed. For example, there is currently about 460 million tonnes (Mt) of copper in use globally – for energy transport, buildings and appliances. Copper can be recycled indefinitely, so if this stock is recovered and repurposed, it could meet about 40% of total supply required for the energy transition [9]. This saves us from needing to mine all materials from the earth – a more costly, risky, and energy-intensive process.

In time, we can also develop closed loops for the critical minerals for clean energy technologies [10]. For EV lithium-ion batteries, it is already the case that recycling can achieve >90% extraction of lithium, cobalt, nickel, and manganese [11]. Best-in-class recovery rates are as high as >99.6% for cobalt and nickel, and >95% for lithium [12].
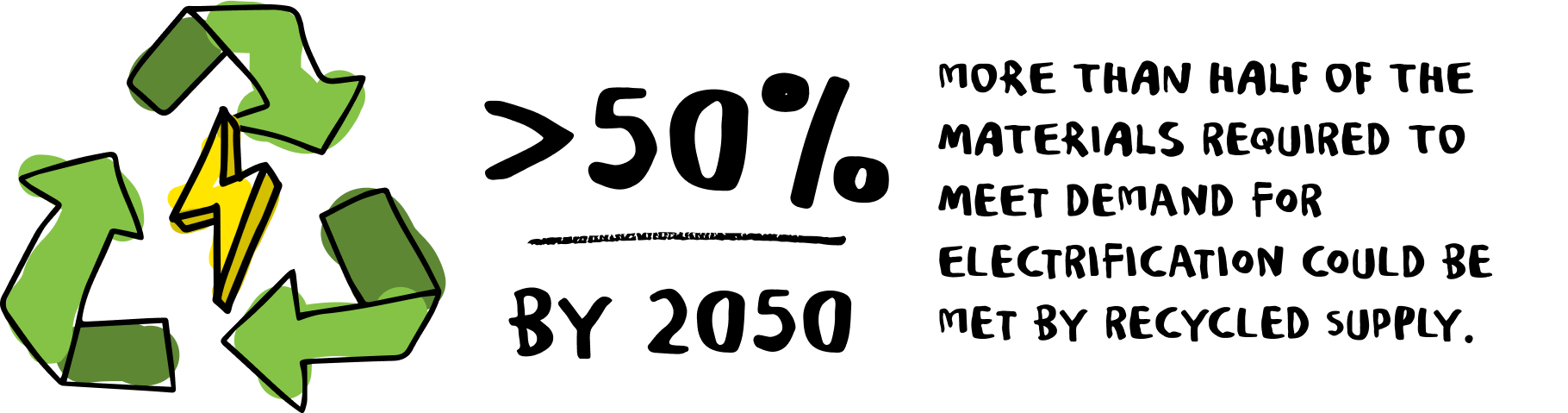
Consequently, the Energy Transitions Commission estimates that, by 2050, more than half of the demand for materials like cobalt, graphite, and lithium could be met by recycled secondary supply [13]. Others, like Rocky Mountain Institute (RMI), expect this to occur even earlier [14].
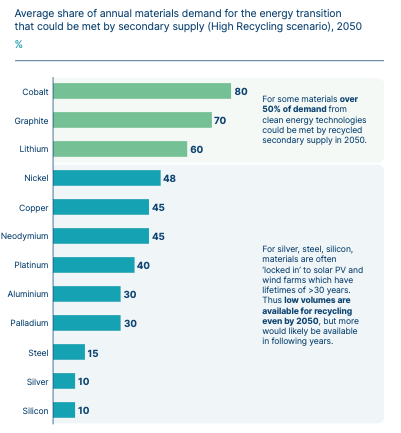
In the meantime, a preemptive strategy of storing, salvaging and re-using retired assets, including lithium-ion batteries, will put us on-track for greater self-sufficiency [15]. In New Zealand, we estimate that the electrification of our private vehicle fleet will involve importing about 760,000 tonnes of battery-related materials, including 100,000 tonnes of aluminium, 150,000 tonnes of nickel, 75,000 tonnes of copper, 45,000 tonnes of manganese, and 35,000 tonnes each of cobalt and lithium. We need to make sure this precious material gets back to manufacturers.
There are already early movers in New Zealand, such as Phoenix Metalman Recycling, which is recycling 98% of solar panel materials to recover aluminium, magnesium, silicon, iron, lead, cadmium, argentum, glass, fibreglass and polyurethane.

But to catch a glimpse of a more systematic approach to circularity, we should look across the ditch to Australia. Victoria, South Australia, Queensland and the ACT have already banned the disposal of solar panels into landfill, with Western Australia to follow suit in 2024 [16]. This both incentivises and stabilises the supply of end-of-life modules for innovators like PV Industries in Sydney and Re-Source in Melbourne, which are recycling around 100 tonnes of silicon-based solar panels per year.
Scale makes this more economically viable. More than one-third of Australian households have rooftop solar, compared to less than 3% in New Zealand [17]. Thus, the volume of potential waste flows in Australia is significantly larger. A recent study estimated that, by 2035, Australia will have 1 million tonnes of solar panels that have reached their end of life – which would be worth over $1 billion as recovered material [18].
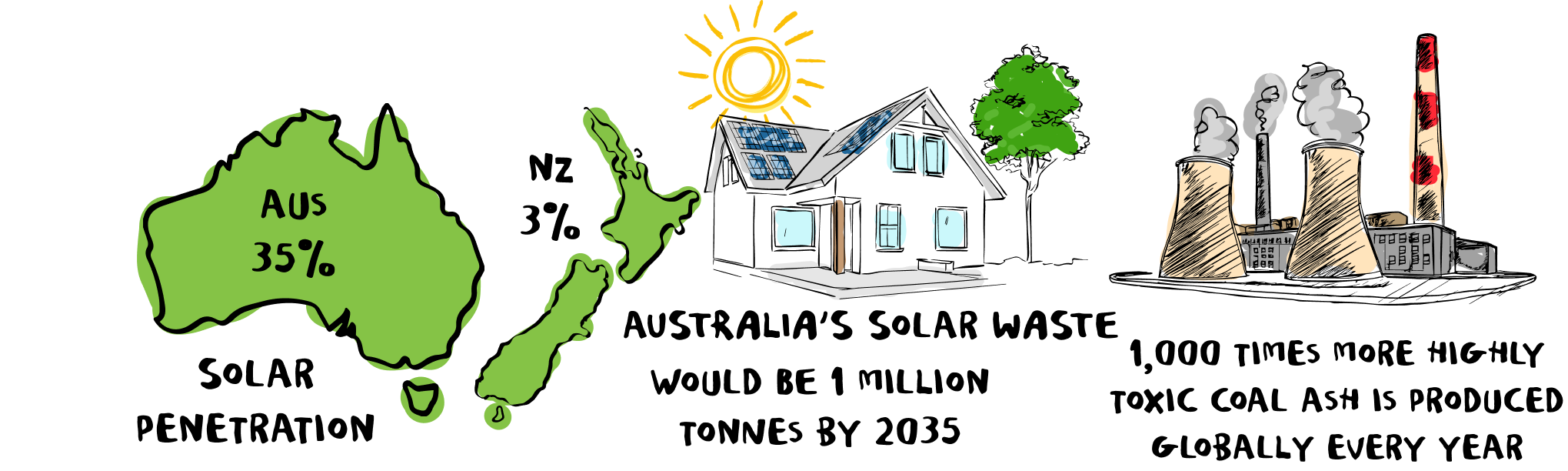
What should New Zealand do? Do we invest in onshore recycling capacity [19], or latch onto the closed loops of other countries? If the latter, whose loop do we join, and what are the geo-political implications of doing so? We return to these questions at the end.
Smart materials
Smart materials are those that do not harm the health of people or environments. They might be grown rather than extracted, sequester carbon during production, biodegrade after use, or even be used indefinitely. In the energy sector, there are significant opportunities to create technologies that utilise biological materials to reduce harm throughout the lifecycle of a product.
A local example is CarbonScape, a New Zealand company which is turning wood chips and sawdust into biographite for use in EV lithium batteries. Consequently, the carbon in woody biomass is effectively stored in products, rather than contributing to global warming. Further, it reduces demand for traditional synthetic graphite which is made by polymerising petroleum molecules, a far more emissions-intensive process.
An offshore example is Modvion, a Swedish company which is creating wind turbines from engineered wood. In 2023, Modvion completed a 105 metre tall tower to power a V90-2.0MW turbine, the tallest such tower in the world. The wood substitutes steel and concrete, both of which are currently emissions-intensive materials. This is an elegant solution for Aotearoa New Zealand, a country with no shortage of either wind or wood.
In solar technology, there are even more fascinating experiments. Solar paint is a family of technologies which, as the name suggests, are applied like regular paint but can generate electricity from the sun. One such technology uses perovskite, one of the most abundant minerals on the planet, which can be sprayed onto buildings, or applied as a thin film onto windows.
Finally, being smart about materials isn’t only about which materials we use, but also which materials we don’t. A common cause for concern is per-and poly-fluoroalkyl substances (PFAS). These so-called ‘forever chemicals’ do not break down in the environment, instead accumulating in the bodies of fish, wildlife and humans with potential health impacts. PFAS are sometimes used for resistant coatings and adhesives, so it is often presumed that they are used in solar PV panels. However, this is not ordinarily the case, because safer alternatives have already been developed and commercialised (e.g. silicone polymers) [20]. For safety’s sake, New Zealand should simply prohibit the production and importation of all assets that use PFAS, a move that wouldn’t impede the energy transition.
Circular Business Models
Circular business models create economic value within natural resource limits, generating jobs and enabling customers to do more with less. The OECD has identified five models: (1) circular supply, (2) resource recovery, (3) product life extension, (4) sharing of assets, and (5) product-as-services [21].
The first two models we have touched on already. Circular supply refers to businesses which use renewable resources, such as wood instead of steel, or solar power instead of fossil fuel. Resource recovery refers to closed loop systems which make products from secondary materials, such as new batteries from old lithium, or solar panels from recovered metals. Two of New Zealand’s most successful innovators, LanzaTech and Mint Innovation, are circular entrepreneurs of this kind.
Product life extension refers to companies that create value by re-using or re-purposing products, effectively delaying their end-of-life. Instead of closing loops, these business models ‘slow the loop’, prolonging the usage of products before its components are recycled. A good example is the re-use of EV batteries, which typically retain 70–80% storage capacity at the end of their guarantee, still adequate for static storage uses like a home battery [22].
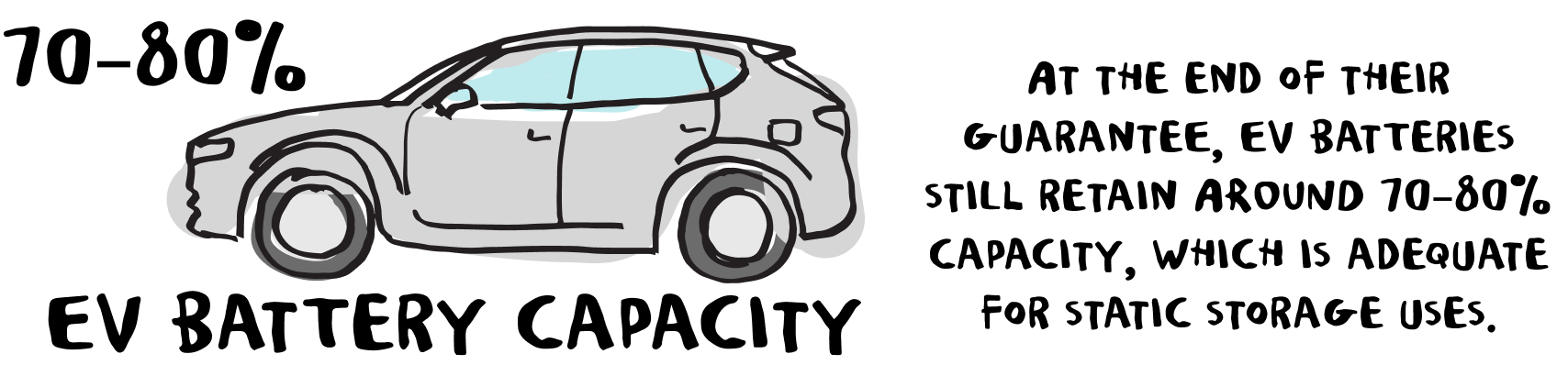
Many companies around the world are exploring re-use opportunities [23] – including in New Zealand:
- Counties Energy partnered with Melbourne-based Relectrify to deliver a second-life battery pilot project, which repurposed EV batteries to create a 120kWh second-life battery system. The first unit, the Berm Battery, was deployed in Mercer, Waikato, for recharging EVs [24].
- Ewi Energy is also creating second-life battery systems for emergency back-up, EV charging and microgrid storage. Customers can buy a WATT BOX which includes two 11kW EV chargers, along with a Starlink satellite connection for internet access. Although Ewi Energy is selling to other businesses, it may offer its products more widely as demand increases.
Another example of product life extension is the electrification of machines that once depended on fossil fuels, also known as e-retrofitting. One example is UK-based Lunaz which installs clean-air powertrains in trucks and industrial vehicles, which retains up to 90% of the materials and embedded carbon in the vehicle. Closer to home, Tranzit was the first in the Southern Hemisphere to convert diesel buses to electric, including a retro-fitted double-decker which was trialled in Auckland [25]. Even closer still, Rewiring’s Mike Casey is upcycling a Toyota Hilux, that stalwart of rural mobility, with a second-hand Nissan Leaf motor. In all these cases, electrification gives a new lease on life for assets which might otherwise have gone to the junkyard.
Sharing of assets refers to business models which increases the utilisation of assets beyond a single user. Simply put, it’s about sharing things. We already see this in shared e-scooter and EV schemes, or electric charging stations. Rather than everyone needing to own these assets, lots of people can share them in a managed, organised way. It also incentivises the manufacturers to build assets that are durable and recyclable.
Finally, products-as-services refer to business models that provide access to products on a rental basis, rather than ownership. This helps homes to electrify without paying the upfront costs of electric machines. For example, Electrolux has piloted a scheme in Sweden and China where customers pay to use appliances via a subscription, rather than owning the appliances outright. Throughout the trial, Electrolux sought to encourage the reuse of resources, efficient energy usage and support product lifetime extension.
Another example is Energy-as-a-Service (EaaS) schemes where customers pay a subscription fee in return for bundles of energy services [26]. A local example is SolarZero which retains ownership of the solar systems it lends out. At a larger scale, Schneider Electric has installed advanced microgrids overseas on a subscription basis [27]. In both cases, the provider carries the upfront capital costs, as well as ongoing risks of continued ownership and maintenance. This creates incentives for providers to use energy assets that are durable, modular and reparable.
Regenerative Behaviours
What about the role of humans in the circular economy? Regenerative behaviours are required to continually care for and sustain our world. In Aotearoa New Zealand, the idea of circular practice resonates strongly with mātauranga Māori. As Johnnie Freedland has said, the ‘whakapapa centred logic’ of Māori culture has a circular quality, which contrasts with the linear mentality that pervades our take-make-and-waste culture [28].
The best-known regenerative behaviour is recycling, where we – as consumers – take the time to sort our end-of-life products and send them where materials can be recovered. But there is much more we can do, not merely as consumers, but also as role models, employees, investors and citizens [29]. We can encourage our work colleagues to take a circular approach to energy and electronics. We can use our spending power to support start-ups that are innovating in circular energy, like second-life batteries. And in the places we live, we can lend our support to community energy projects which (among other things) use resources more effectively, and maximise the value of every energy asset by orchestrating supply and demand across the community [30].
We can also avoid degenerative behaviour – that is, exploitative and harmful relations with ecosystems and communities. This requires facing up to the negative impacts of the fossil energy system: its legacy of destructive mining, human rights violations and environmental spillovers. It also involves recognising that renewable energy is not free from such challenges either, which we cannot afford to reproduce as demand for renewable energy technologies scales up. As the Just Transition Finance Lab put it recently, reducing the negative impacts of mining is ‘one of the most crucial tasks ahead for the just transition: avoiding a trade-off between the expansion of mineral production for global climate action and the achievement of positive local outcomes’ [31]. This requires us to keep tabs on how we source our materials for electrification.
Network Participation & Embedded Intelligence
Energy innovation is already making the electricity sector more networked and intelligent. Peer-to-peer trading, or multiple trading relationships, enable communities to better utilise the electricity that they generate [32]. Also, a blossoming of new technologies – including smart meters, smart grids, open energy data platforms, Internet of Things (IoT) – are making our energy systems more efficient and responsive [33].
However, there are also opportunities to harness these digital capabilities for the circular economy [34]. Data utilisation can increase waste reduction and the traceability of materials and parts, which not only enables circularity but also the overall efficiency of supply chain operations. This can help the energy sector to turn supply chains into supply circles [35], where spare parts can be efficiently located, allocated and transported for re-use. Data also helps decision makers to plan ahead; for example, RMI’s Battery Circular Economy Initiative (BCEI) Dashboard provides stakeholders with insights on supply chains in the present and future.
As a technology-taker, New Zealand cannot afford to neglect the digitalisation of supply chain management. Companies and countries are adopting targets for circularity, not least of which is the world’s clean tech colossus, China. It already has 2025 targets to increase resource productivity by 20% compared to 2020, and to increase the output value of its resource recycling industry [36]. Digitalisation will help China and other technology providers to keep track of resource flows, and to hold countries like New Zealand accountable for returning re-usable materials into the global economy.
Where to from here?
If we stumble into the energy transition with a linear mindset, we will cause harm to people and the planet. Machines will clutter up landfills. People will lose confidence in the energy transition, appalled by the waste and costs of electrification. And demand for primary resources will be higher than it needs to be, exposing manufacturers and consumers to supply chain bottlenecks and higher prices.
But we can avoid this situation – and we have many incentives to do so. A far-sighted, preemptive approach to circularity can minimise energy-related waste, shift material dependencies from mining to recycling, and strengthen public support for renewable energy. Furthermore, it will unleash a new wave of entrepreneurs and innovators – like Mint Innovation, CarbonScape and others – who are finding new ways to create value from end-of-life assets.
Here are three big ideas which will help to unlock the full potential of a circular energy transition.
First, Aotearoa New Zealand needs a circular energy roadmap which coordinates the efforts of government, industry and community. The energy transition is a predictable challenge – we know it’s happening – so there is no excuse not to prepare for it. The New Zealand Government’s Draft Mineral Strategy commits to accelerating the circular economy for critical minerals in the next three years [37], but only in general terms.
In Australia, by contrast, an industry-led roadmap on solar panel waste was published in March 2024 [38], which recommends using multiple levers that include awareness-raising campaigns, optimisation of waste logistics, R&D funding for circular innovation, national standards for re-use and recycling, and investment into the infrastructure of collection centres and waste management facilities [39]. And overarching all this is a product stewardship framework, which is the next big idea.
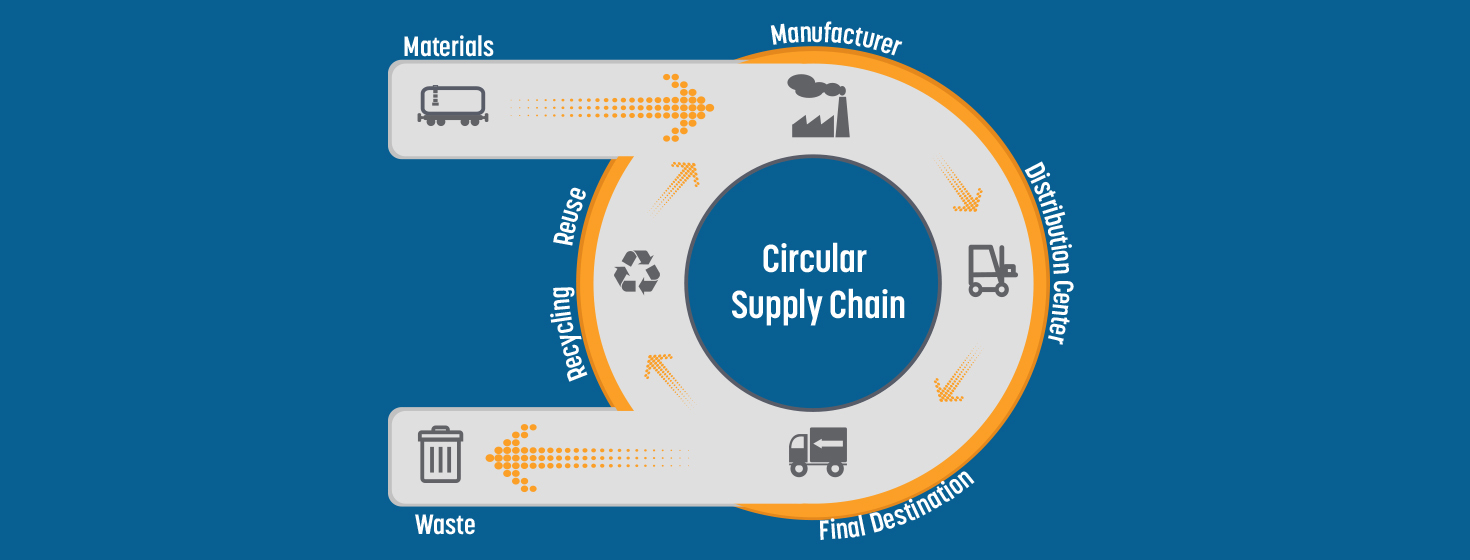
Second, Aotearoa New Zealand needs to embolden its culture of product stewardship, which shares responsibility for the management of end-of-life products among all parties involved in producing, selling, using and disposing of them. The New Zealand Government has legislative powers in the Waste Minimisation Act 2008, which includes e-waste from solar panels and large batteries among other ‘priority products’ [40].
The Ministry for the Environment plans to improve this law for better e-waste product stewardship in 2024/2025 [41]. However, it might consider a more robust approach through Extended Producer Responsibility (EPR) which encourages businesses to take full responsibility for waste minimisation across the lifespan of their products. This incentivises businesses to invest upfront in extending the last legs of products and technologies, which delays and minimises the costs of end-of-life management. It also encourages businesses to invest in modular design and decomposability, which improves the economics of repair, reuse and recycling of solar panels, batteries and other technologies.
Third and finally, we need to support innovation and entrepreneurship in circular energy systems. The vision of electrifying and recirculating (nearly) everything is a practical one, a moonshot we could plausibly achieve. It could be a source of innovation and prosperity, as well as avoided costs, but only if we invest now in energy, waste and digital infrastructure. This will involve cross-sector partnerships and collaborations, and mobilising public and private investment into research, development and deployment (RD&D).
One such model is the Plastics Innovation Fund which supports organisations to experiment with plastic circularity. Critically, this needs to be strategic and targeted, taking a challenge-led approach which strives to tackle the problem of e-waste by intentionally pursuing circularity in energy systems [42]. This might include public procurement to support early-stage circular innovation to boost the scale and competitiveness of new technologies and practices. Similarly, innovation prizes could be used to solve specific circularity challenges, such as the development of alternative materials, or modular design that makes repairs and recycling easier. This could supplement the circular ambitions in the New Zealand Government’s mineral strategy.
All this is possible, but we cannot take it for granted. We must choose to seize these opportunities to bring renewable energy and the circular economy together.
.png)
1) The Ellen MacArthur Foundation (n.d.). The Circular Economy in Detail.
2) Lacy, P., Long, J. and Spindler, W., (2020). The Circular Economy Handbook. Palgrave Macmillan.
3) Circle Economy Foundation (2024). The Circularity Gap Report 2024.
4) Yadav, V. K., Modi, T., Alyami, A. Y., Gacem, A., Choudhary, N., Yadav, K. K., ... & Jeon, B. H. (2023). Emerging trends in the recovery of ferrospheres and plerospheres from coal fly ash waste and their emerging applications in environmental cleanup. Frontiers in Earth Science, 11, 1160448.
5) Jeff Turentine (2019). Coal Ash Is Hazardous. Coal Ash Is Waste. But According to the EPA, Coal Ash Is Not ‘Hazardous Waste’. Natural Resources Defense Council (NRDC).
6) Mirletz, H., Hieslmair, H., Ovaitt, S. et al. (2023). Unfounded concerns about photovoltaic module toxicity and waste are slowing decarbonization. Nature Physics 19: 1376–1378.
7) Van Opstal, W., & Smeets, A. (2023). Circular economy strategies as enablers for solar PV adoption in organizational market segments. Sustainable Production and Consumption, 35, 40-54.
8) For an economy-wide overview, see Aurecon, thinkstep-anz and Sustainable Business Network (2024). Mapping Emissions and Waste Stream Profiles, and Opportunities for Achieving Net-zero Circular Advanced Manufacturing.
9) Energy Transitions Commission (2023). Material and Resource Requirements for the Energy Transition.
10) The closing of battery loops will take time, because secondary supply of minerals only becomes available as today’s batteries reach end-of-life. This will be delayed further by improved battery life and re-use models. Energy Transitions Commission (2023). Material and Resource Requirements for the Energy Transition.
11) Zhang, J., & Azimi, G. (2022). Recycling of lithium, cobalt, nickel, and manganese from end-of-life lithium-ion battery of an electric vehicle using supercritical carbon dioxide. Resources, Conservation and Recycling, 187, 106628.
12) Daan Walter, Will Atkinson, Sudeshna Mohanty, Kingsmill Bond, Chiara Gulli, Amory Lovins (2024). The Battery Mineral Loop: The path from extraction to circularity. Rocky Mountain Institute (RMI).
13) Energy Transitions Commission (2023). Material and Resource Requirements for the Energy Transition.
14) RMI (2024). Battery Circular Economy Initiative Dashboard. Rocky Mountain Institute (RMI).
15) Juhi Shareef (2019). New energy futures paper: Batteries & the circular economy. Vector Ltd. https://blob-static.vector.co.nz/blob/vector/media/vector/vector_new_energy_futures_paper_batteries.pdf
16) Keep Australia Beautiful (2024). Recycling solar panels: Chipping away at our mountain of waste.
17) Electricity Authority (2024). Installed distributed generation trends. www.emi.ea.govt.nz/r/sbdd4
18) Rong Deng, Verity Tan, Chence Niu, Renate Egan (2024). Scoping study: Solar panel end-of-life management in Australia. Australian Centre of Advanced Photovoltaics (ACAP).
19) See, for instance, the proposal by The Southern Initiative and The Western Initiative (2021). Creating shared prosperity through the circular economy: Building Māori & Pasifika economic resilience through a regenerative and circular enterprise movement. Auckland Council.
20) Annick Anctil (2020). Facts about solar panels: PFAS contamination. ‘Clean Energy in Michigan’ Series, 12. Michigan State University.
21) OECD (2019). Business Models for the Circular Economy: Opportunities and Challenges for Policy. OECD.
22) Liz Najman (2024). How Long Do Electric Car Batteries Last? Recurrent.
23) Box of Energy in Sweden has re-used EV batteries for home energy systems, installing silver cabinets about the size of a large refrigerator, each using 20 battery modules recovered from Volvo hybrid cars. They store energy from rooftop solar panels to run the elevators and lights in common areas.
24) Counties Energy (2024). Counties Energy repurposes end of life EV batteries to recharge new EV cars.
25) Todd Niall (2022). Southern Hemisphere's first converted electric double-decker begins Auckland trial. Stuff.
26) IEA (2022). The Potential of Digital Business Models in the New Energy Economy. International Energy Agency (IEA).
27) Schneider Electric (2019). The Evolution of Energy-as-a-Service.
28) Daniel Christian Wahl and Johnnie Feedland (2020). ‘Circular & square systems thinking’ — a Maori perspective on regeneration. Medium.
29) Nielsen, K. S., Nicholas, K. A., Creutzig, F., Dietz, T., & Stern, P. C. (2021). The role of high-socioeconomic-status people in locking in or rapidly reducing energy-driven greenhouse gas emissions. Nature Energy, 6(11), 1011-1016.
30) Ara Ake (2023). Community Energy: How to Guide.
31) Antonina Scheer and Nick Robins (2024). Unjust minerals: investing in the changes needed for a just transition in the mining sector. Just Transition Finance Lab.
32) See, for example, the Multiple Trading Relationship (MTR) trial by Kāinga Ora and Ara Ake (2024). Kāinga Ora Multiple Trading Trial.
33) IRENA (2019). Innovation Landscape for a Renewable-Powered Future: Solutions to Integrate Variable Renewables. International Renewable Energy Agency (IRENA).
34) Eva Barteková and Peter Börkey (2022). Digitalisation for the transition to a resource efficient and circular economy. OECD Working Paper.
35) Nea Laine, Benjamin Findlay and David Stroud (2023). How data standards can transform supply chains into supply circles. World Economic Forum (WEF).
36) Alexander Chipman Koty (2021). China’s Circular Economy: Understanding the New Five Year Plan. China Briefing. Dezan Shira & Associates.
37) MBIE (2024). A draft Minerals Strategy for New Zealand to 2040. New Zealand Government.
38) Rong Deng, Verity Tan, Chence Niu, Renate Egan (2024). Scoping study: Solar panel end-of-life management in Australia. Australian Centre of Advanced Photovoltaics (ACAP).
39) Other countries are exploring different policy mixes, including subsidies to incentivise better waste management. In the US, the Environmental Protection Agency (EPA) oversees the Responsible Appliance Disposal (RAD) programme, which pays owners to recycle old, inefficient appliances like refrigerators and freezers. This incentivises people to upgrade to newer, more efficient appliances and keeps old appliances out of landfill.
40) Alice Logan (2024). When the sun sets on solar panels: End of life solutions. Minter Ellison.
41) Ministry for the Environment (2024). Priority product stewardship. New Zealand Government.
42) Ingrid Burkett (2023). Challenge-led innovation: Organising for Systems Innovation at Scale. Griffith Centre for Systems Innovation.